Abstract
Background:
Interleukin-4 (IL-4), as the most prominent anti-inflammatory cytokine, plays an important role in modulating microglial activation and inflammatory responses in Alzheimer’s disease (AD), a chronic inflammatory disorder.Objectives:
The current study aimed to develop a new recombinant Adeno-associated viral (rAAV) vector that delivers IL-4 and then assess the counterbalancing effect of the new construct along with recombinant IL-4 (rIL-4) protein in in-vitro models of AD.Materials and Methods:
The rAAV-IL4 was originally prepared and then employed along with rIL-4 protein to counter Amyloid β (1-42)-induced proinflammatory cytokines in a primary microglia cell culture and the B92 rat microglia continuous cell line, using relative Real-Time PCR assay.Results:
Aβ (1-42) stimulated the production of the proinflammatory cytokines IL6, IL1β, TNFα, and IL18 in both the primary microglia cell culture and the B92 cell line. Both the rAAV-IL4 construct and the rIL-4 protein were found to inhibit production of the most important Aβ (1-42)-induced proinflammatory cytokine mRNAs in the two types of cells with different patterns.Conclusions:
It seems that the new construct can serve as an appropriate option in the modulation of Aβ-induced proinflammatory cytokine gene expression and microglia activation in patients affected by AD.Keywords
Alzheimer Disease Adeno-Associated Viral Vector Amyloid β (1-42) Proinflammatory Cytokines IL-4 Primary Microglia Cell B-92 Cell Line
1. Background
Recombinant Adeno-associated viral (rAAV) vector is one of the most desirable vehicles for gene delivery to the central nervous system (CNS), post-mitotic tissues, and in immune-privileged situations (1).
Adeno-associated virus is a small DNA virus having a 4.7 kb genome and belonging to the Parvoviridae family, the Parvovirinae subfamily, and the Dependovirus genus. Currently, more than 100 isolates of AAV species from various animals have been identified (2). Diverse tissue tropism; very mild immune reactivation; no relation to any well-known disease; genome integration; and long-term, stable gene expression make this virus an appropriate vector to deliver trans-complementary genes to various problematic organs and tissues such as muscle, liver, eye, and CNS (2, 3). Recently, AAV viral vector has been used to investigate various neurological disorders such as Alzheimer’s disease (AD) (4, 5), Parkinson’s disease (PD) (6), and spinal cord injury (7).
Alzheimer’s disease is the most frequent cause of dementia in the aging population. It is expected that the number of those with AD will increase by approximately 50% by 2050 (8, 9). Brain atrophy and neuronal-cell loss are the main hallmarks of this disease (10, 11) and are associated with two significant neuropathological features: neurofibrillary tangles (NFTs) and neuritic plaques (10). Neurofibrillary tangles are inter-neuronal, dense bundles of hyper-phosphorylated tau protein, and neuritic plaques are intra-neuronal masses surrounding a fibrillar β-amyloid core. Activated microglia are consistently associated with these plaques, and it is believed they are stimulated by Amyloid-β (Aβ). Amyloid-β fragments serve as immunoglobulin (Ig) and activate inflammatory mechanisms via alternative pathways, while the classic inflammatory response is absent (12).
The activation of microglia associated with neuritic plaques leads to the production of proinflammatory cytokines, inflammatory markers, and neurotoxic mediators, including MHC-I and II, TNF-α, IL-6, IL- 1α, IL-1β, B7-1, B7-2, IFN-α, GMCSF, type B of IL-8 receptor, MCP-1, iNOS, NO, CD40, and others (10, 13-15).
Given the established evidence demonstrating the role of the inflammatory process in AD pathogenesis, researchers are investigating the use of anti-inflammatory drugs as a treatment option for the disorder (5, 16). IL-4, the most popular anti-inflammatory cytokine, has a significant inhibitory role in the expression and release of the proinflammatory markers, including IL-1, IL-6, IL-8, and TNF-α, and stimulates the synthesis of IL-1 receptor antagonist (IL-1ra), which blocks the action of IL-1α and IL-1β (17-20). In addition, IL-4 expression results in marked expression of the scavenger receptor CD36, the Amyloid-β degradation enzyme neprilysin (NEP), and insulin-degrading enzyme (IDE) (19).
2. Objectives
The present study aims to construct a unique rAAV vector that expresses IL-4, which counters AD and its deleterious immune reactivated pathways. For this purpose, we generate high-transduction efficiency rAAV that encodes IL-4 and model its effect for the first time in vitro, evaluating its inhibitory effects on the inflammatory process induced by Aβ (1-42) in primary and continuous cell lines using real-time PCR assay.
3. Materials and Methods
3.1. RNA Extraction, cDNA Synthesis, and Cloning of the PCR Product
Adult male Sprague Dawley rats weighing 175 - 200 g were procured from Shiraz University of Medical Sciences Animal Center and housed in the Animal House of Nemazi Hospital, Shiraz, Iran. The tail artery was cut to drain the oxalate blood. Total RNA was isolated from fresh peripheral blood mononuclear cells (PBMCs) with the use of QIAamp RNA blood mini kits (QIAGEN; Valencia, CA), according to the instructions provided by the manufacturer. Appropriate primers for the IL-4 gene were designed based on the published RNA sequences. The rat IL-4 primer sequences were as follows: IL-4 upstream: 5'-CGCGGATCCCTGACTGTAGAGAG-3' and IL-4 downstream: 5'-CCCGATATCTTTCAGTGTTGTGAGCGT-3'. The expected length of the PCR products was 444 bp.
The RNA was reverse-transcribed and amplified by PCR with a one-step RT-PCR assay in a total volume of 50 µL with 0.2 mg of total RNA and 1 mM each of upstream- and downstream-specific primer corresponding to the interested sequences. Reactions were incubated at 48°C for 45 minutes, heated to 94°C for 2 minutes, and cycled according to the following parameters: 94°C for 30 seconds, 52°C for 1 minute, and 72°C for 2 minutes for a total of 40 cycles. After 40 cycles, a 20-minute final extension at 72°C was performed.
The cloning of the IL-4 cDNA into pTZ57R/T was performed as described by the supplier (InsTAclone PCR cloning kit; Fermentas; USA). Then, it was transformed into the Escherichia coli DH5α. The transformants were chosen on LB-Amp (50 mg/mL) agar plates supplemented with IPTG and X-Gal. Desired constructs were confirmed by PCR, restriction enzyme analysis, and, finally, DNA sequencing (MWG Co; Germany) using universal M13 PUC primers.
3.2. Generation, Purification, and Quantification of rAAV-IL4 Gene Expression
The pTZ57R/T-IL4 was digested with BamHI/EcoRI and subcloned into corresponding restriction sites of pAAV-IRES-Hygro (CMV promoter; Cell Biolab; San Diego, CA). To prepare the rAAV-IL4, the desired construct (pAAV-IRES-Hygro-IL4) was cotransfected with the pAAV-DJ/8 Vector (containing AAV-2 Rep, AAV-DJ/8 Cap, and ampicillin resistance genes) and pHelper Vector (containing Adeno E2A, Adeno E4, and Adeno VA) into the AAV-293 cell line, using Lipofectamine 2000. The rAAV-IL4 vector was purified using ViraBin AAV purification kits (Cell Biolab; San Diego, CA). The genome copy number was assessed by QuickTiter AAV quantitation kits (Cell Biolab; San Diego, CA).
3.3. Primary Microglia and the B92 Cell Culture
Primary cultures of microglia were provided from Wistar rat pups 1 - 2 days old. Briefly, in aseptic conditions, the skull (without any brain squish) was removed and 3 layers of meninges were stripped from the cortices in a sterile Petri dish containing pre-chilled Hank’s buffer salt solution (HBSS). Dissected cortex tissue was transferred into 50 mL of sterile, ice-cold, conical tubes containing 10 mL of dissociation media (Ca+2 and Mg+2 - free MEM + penstrep + 0.25% trypsin-EDTA) on ice. Maintaining the cold-chain condition, cultured media was replaced with 12 mL of dissociation media as often as possible, and the cortex was then gently pulverized and homogenized 10 - 15 times using a fire-polished 10-mL, glass, Pasteur pipette and, finally, stirred for 10 minutes at 37°C in the laminar-flow hood. Once the homogenized suspension reached 50 mL high glucose DMEM + 10%, inactivated FBS + penstrep was added and centrifuged 1000 × g for 10 minutes. The cells were resuspended in growth medium, followed by seeding of 1.5 × 106 cells in 25 cm2 flasks coated with poly-l-lysine and grown at 37°C in a 95% humidified, 5% Co2 cell-culture incubator. Growth medium was replaced 7 days after mixed-glial cell culture occurred. Approximately 2 weeks after initial plating, when astrocytes reached 100% confluence, microglia, growing as a small, rounded cell on the top layer, was detached from the astrocytes bed by gentle shaking, and then, 2 × 105 viable cells/well were reseeded in 24-well culture plates.
The B92 cells were prepared from the Pasteur Institute of Iran and maintained in DMEM + 10% FBS + penstrep at 37°C in a 95% humidified, 5% Co2 cell-culture incubator, and 3 × 105 cells were plated in 24-well culture plates.
3.4. Treatment of Primary Microglia and B92 Cells With Aβ in the Presence or Absence of rAAV-IL4 and rIL-4 Protein
In the first experiment, to evaluate Aβ (1-42)-induced proinflammatory cytokine expression patterns, one 8-series of 3 × 105 of B92 cells and 2 × 105 of primary microglia cells were grown in a 500-λ medium containing 10% FBS in 24-well plate tissue-culture plates for 24 hours. After incubation overnight, cells were incubated with 500 λ of 10% FBS growth media containing 10 µM of Aβ (1-42) for 0, 1, 2, 4, 8, 16, 24, and 48 hours.
In another experiment to assess the counterbalancing effect of IL-4 against Aβ (1-42)-induced proinflammatory cytokines, both B92 cells and primary microglia cells were grown, following the same method as used in the above experiment, for 24 hours. The following morning, the medium was replaced with 200 λ of fresh medium containing 10% FBS and 150 λ of culture medium containing 10 nM of recombinant rat IL-4 protein or 1010 pfu of rAAV-rat-IL4 construct. Both were incubated for 1 hour, and then 150 λ of culture medium containing 34 µM of Aβ (1-42) was added to the plates and incubated for 0, 1, 2, 4, 8, 16, 24, and 48 hours.
3.5. Real-time Quantitative PCR to Detect Changing of Cytokine Gene Expression
In both series of the above experiments, the proinflammatory mRNA expression levels were quantified using the SYBR green PCR assay system. The reaction mixtures (20 λ) consisted of 1X power SYBR green PCR master mix buffer (Applied Biosystems; UK), 400 nM of each primer, and 200 ng of cDNA. Table 1 shows the primer sequences of the desired proinflammatory cytokines. The reaction conditions were an initial 2 minutes at 50°C and 10 minutes at 95°C, followed by 40 cycles of 15 sec at 95°C and 30 seconds at 65°C.
Sequences of Forward and Reverse Primers for Quantitative PCR
Primer Name | Forward Primers | Reverse Primers |
---|---|---|
rat IL-6 | 5'CGAGCCCACCAGGAACGAAAGTC3' | 5'CTGGCTGGAAGTCTCTTGCGGAG3' |
rat TNF-α | 5'GACCCTCACACTCAGATCATCTTCT3' | 5'TGCTACGACGTGGGCTACG3' |
rat IL-1β | 5'CCCTGCAGCTGGAGAGTGTGG3' | 5'TGTGCTCTGCTTGAGAGGTGCT3' |
rat IL-18 | 5'CAGACCACTTTGGCAGACTTCACT3' | 5'GGATTCGTTGGCTGTTCGGTCG3' |
rat β2M | 5'CGTGATCTTTCTGGTGCTTGTC3' | 5'TTCTGAATGGCAAGCACGAC3' |
3.6. Statistical Analysis
The results were analyzed by SPSS for windows systems (Version 16.0, 2007; SPSS Inc; Chicago, IL). A two-sided Student’s t test was applied to analyze the RT PCR results. Values were considered significant when P < 0.05. The results were expressed as mean ± SEM.
4. Results
4.1. Preparation of the rat rAAV-IL4
The total PBMCs RNAs of the rats were extracted, and 444 bp of IL-4 cDNA was synthesized. The desired gene was amplified using specific primers and then evaluated through electrophoresis on 2% gel agarose (Figure 1). The IL-4 cDNA was cloned in pTZ57R/T cloning vector and then evaluated by restriction-enzyme analysis and sequencing (Figure 2). The EcoRI/BamHI digested construct was subcloned into the corresponding restriction site of the AAV plasmid. The presence of a desired gene was confirmed by restriction-enzyme analysis.
Rat IL-4 cDNA Gel Electrophoresis
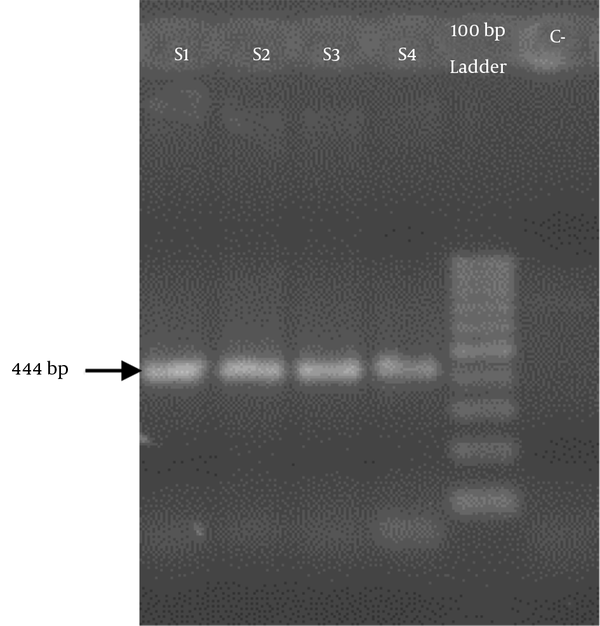
Restriction Enzyme Analysis of pTZ57R/T-rat-IL4
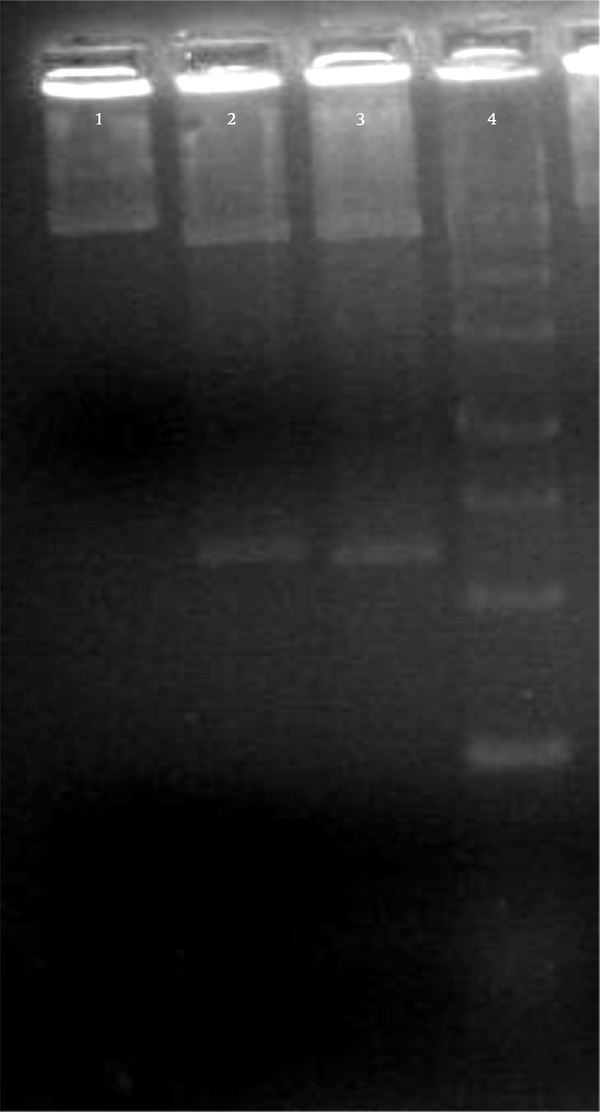
4.2. mRNAs’ Proinflammatory Cytokine Patterns Induced by Aβ (1-42)
The current study investigated the mRNA expression level of the specified proinflammatory cytokines, IL1-β, IL-6, TNF-α, and IL-18, in Aβ (1-42)-induced rat primary microglia cell culture and the B92 cell line over a 48 hours period.
In the primary microglia cell culture, the greatest fold changes occurred in the expression of IL1-β, TNF-α, IL-6, and IL-18 mRNAs sequentially, while the primary raised cytokine was TNF-α within 4 hours after Aβ (1-42) treatment (Figure 3A). In the B92 cells, TNF-α increased remarkably, followed by IL-1β, IL-6, and IL-18 (Figure 3B). It is notable that the expression level of proinflammatory cytokines in microglia primary cell cultures was generally higher than in the B92 cell line.
Changing Cytokine Gene-Expression Profiles During Specified Times After Induction by 10 µM of Aβ (1-42)
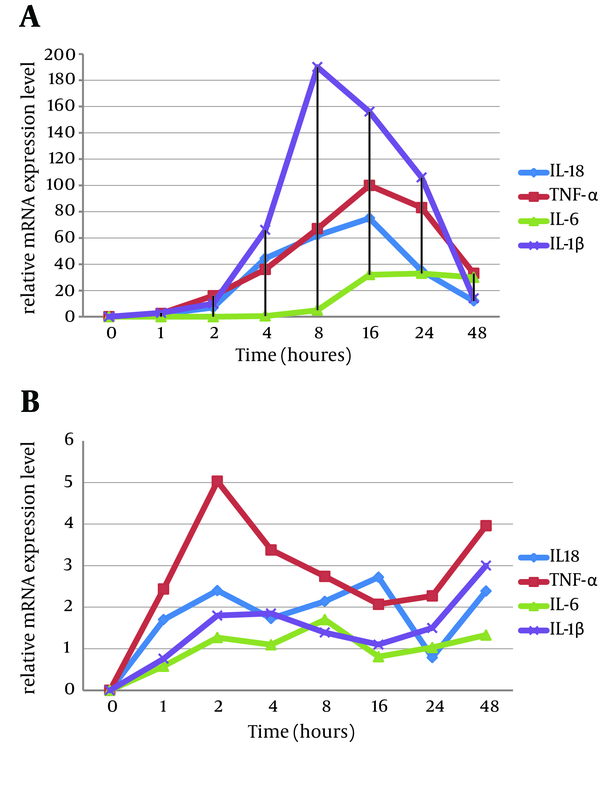
4.3. Inhibitory Effect of rIL-4 and rAAV-IL4 on Proinflammatory Cytokine Production in the B92 Cell Line and the Primary Microglia Cell Culture
The present study surveyed the modulatory effects of both recombinant IL-4 protein and rAAV that expressed IL-4 on the production of the selected proinflammatory cytokines in both primary microglia and the B92 cell line. Recombinant IL-4 protein down-regulated the expression of Aβ-induced IL-1β, IL-6, TNF-α, and IL-18 mRNAs in both cell types, although the TNF-α changes were slight (Figure 4).
Evaluation of the Inhibitory Effects of rIL-4 Protein and the rAAV-IL4 Construct on the mRNA Expression of IL-18, TNF-α, IL-6, and IL-1β Proinflammatory Cytokines
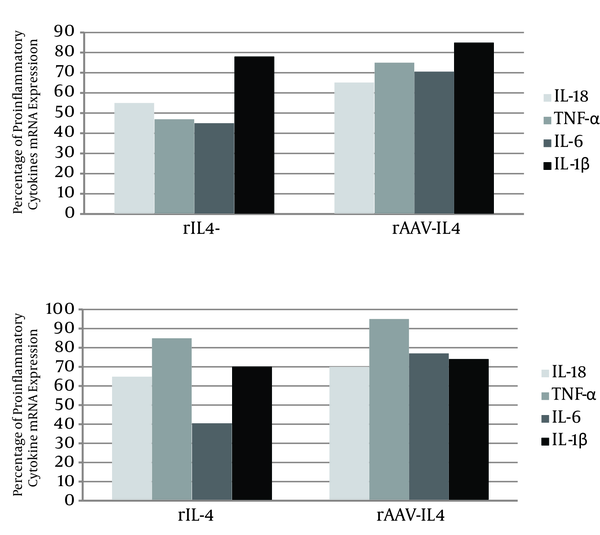
Since the AAV vector used in this study has a relatively high transfection capability in vitro compared to other naive AAV vectors, rAAV-IL4 can inhibit the production of the above proinflammatory cytokines in both the primary microglia cell culture and the B92 cells, except TNF-α and IL-1 mRNA expression, and especially in those B92 cells that were slightly attenuated.
5. Discussion
Various studies have shown the detrimental effects on AD patients’ CNS of proinflammatory cytokines, which are predominantly elicited from microglial cells with chronic exposure to Aβ. The injurious effects of proinflammatory cytokines can lead to neuronal dysfunction and death through mechanisms including the induction of nitric oxide (NO) production (21, 22); long-term potentiation (LTP) of synaptic transmission inhibition, which plays a key role in the formation of memory (23, 24); and reduced hippocampal neurogenesis (25).
Immunotherapy is a newly developed strategy that can help to slow cognitive impairment and reduce the AD-associated lesions (26). Since anti-inflammatory cytokines can protect the CNS from lesions caused by proinflammatory cytokines (27-29), IL-4, as the most prominent anti-inflammatory cytokine, has a wide range of implications for treating AD (4, 26, 27, 30, 31). This cytokine can polarize T-cells and macrophages to Type 2 morphology (Th2 and M2) and accelerate amyloidosis (32). Kiyota et al. showed that AAV-mediated IL-4 can reduce the burden of Aβ (1-42) in vivo (4). Zhao et al. demonstrated that IL-4 can protect the microglia by inhibiting production of free radicals (33). In addition, several other mechanisms of the inhibitory effects of IL-4 have been noted, including its inhibition of formyl peptide receptor (FPR) (34).
This study established that AAV encoding IL-4 the same as rIL-4 protein against Aβ (1-42)-induced IL1-β, IL-6, TNF-α, and IL-18 proinflammatory cytokines in rat primary microglia and in the B92 rat microglia cell line (28). The AAV vector used has a complex library of hybrid capsids from eight different wild-type viruses and has high transduction efficacies, especially in vitro, compared to other wild type serotypes.
Several studies have shown that Aβ (1-42) induces the production of proinflammatory cytokines in vitro (28, 31), in animal labs (4), and in AD patients (26, 35-37), and evidence has shown the contribution of proinflammatory cytokine in the AD development process.
Many studies have shown an elevated level of TNF-α in the serum and cerebrospinal fluid (CSF) of AD patients and have confirmed the potential involvement of this prominent proinflammatory cytokine in AD pathophysiology (31, 35). Tobinick et al. demonstrated that consumption of a TNF-α inhibitor (Etanercept) by AD patients leads to a significant improvement (38). Szczepanik et al. (28) reported that TNF-α was secreted from Aβ (1-42)-induced primary microglia and the THP-1 cell line (39). The current study used quantitative PCR assay to confirm acceleration of the mRNA level of TNF-α in Aβ (1-42)-induced primary and continuous rat microglia cells.
Elevated levels of IL-6 (40, 41) and its contribution to pathophysiology in AD patients has been shown by Cojocaru et al. (41). As shown in Figure 3, the mRNA of this cytokine increased markedly against Aβ (1-42) induction.
Bossu et al. (42) demonstrated an increased IL-18 cytokine level in AD patients and a positive correlation between IL-18 expression and AD-related cognitive impairment (43, 44). The present study demonstrated that Aβ (1-42), like other assessed proinflammatory cytokines (45), can induce IL18 mRNA expression, while its expression can be inhibited by the anti-inflammatory cytokines, either rIL-4 or rAAV-IL4.
In AD-affected patients, the expression of Aβ (1-42)-induced proinflammatory cytokines can cause progressive dementia because of negative neurodegenerative effects. IL-4 not only protects neurons from further injury, but also revives the impaired neurodegenerative lesions (46) by inhibiting IFN-λ priming of microglia through cAMP signaling, with later reduction in impairing proinflammatory cytokines such as TNF-α, IL-1β, and nitric oxide (47).
In conclusion, this study has shown that anti-inflammatory gene delivery by AAV vector plays a significant role in modulating immune response in Aβ (1-42)-induced processes. In addition, it seems that this newly suggested treatment could have implications in other allergic disorders, autoimmune diseases, and transplant rejection.
Acknowledgements
References
-
1.
Howard DB, Powers K, Wang Y, Harvey BK. Tropism and toxicity of adeno-associated viral vector serotypes 1, 2, 5, 6, 7, 8, and 9 in rat neurons and glia in vitro. Virology. 2008;372(1):24-34. [PubMed ID: 18035387]. https://doi.org/10.1016/j.virol.2007.10.007.
-
2.
Wu Z, Asokan A, Samulski RJ. Adeno-associated virus serotypes: vector toolkit for human gene therapy. Molecular therapy. 2006;14(3):316-27. [PubMed ID: 18035387].
-
3.
Gao G, Vandenberghe LH, Wilson JM. New recombinant serotypes of AAV vectors. Curr Gene Ther. 2005;5(3):285-97. [PubMed ID: 15975006].
-
4.
Kiyota T, Okuyama S, Swan RJ, Jacobsen MT, Gendelman HE, Ikezu T. CNS expression of anti-inflammatory cytokine interleukin-4 attenuates Alzheimer’s disease-like pathogenesis in APP+ PS1 bigenic mice. FASEB J. 2010;24(8):3093-102.
-
5.
scarpini E, Schelterns P, Feldman H. Treatment of Alzheimer's disease; current status and new perspectives. The Lancet Neurology. 2003;2(9):539-47. https://doi.org/10.1016/s1474-4422(03)00502-7.
-
6.
Nihira T, Yasuda T, Hirai Y, Shimada T, Mizuno Y, Mochizuki H. Adeno-associated viral vector-mediated gene transduction in mesencephalic slice culture. J Neurosci Methods. 2011;201(1):55-60. [PubMed ID: 21827789]. https://doi.org/10.1016/j.jneumeth.2011.07.010.
-
7.
Ruitenberg MJ, Blits B, Dijkhuizen PA, te Beek ET, Bakker A, van Heerikhuize JJ, et al. Adeno-associated viral vector-mediated gene transfer of brain-derived neurotrophic factor reverses atrophy of rubrospinal neurons following both acute and chronic spinal cord injury. Neurobiol Dis. 2004;15(2):394-406. [PubMed ID: 15006710]. https://doi.org/10.1016/j.nbd.2003.11.018.
-
8.
Crews L, Masliah E. Molecular mechanisms of neurodegeneration in Alzheimer's disease. Hum Mol Genet. 2010;19(R1):R12-20. [PubMed ID: 20413653]. https://doi.org/10.1093/hmg/ddq160.
-
9.
Hebert LE, Scherr PA, Bienias JL, Bennett DA, Evans DA. State-specific projections through 2025 of Alzheimer disease prevalence. Neurology. 2004;62(9):1645. [PubMed ID: 15136705].
-
10.
Benveniste EN, Nguyen VT, O'Keefe GM. Immunological aspects of microglia: relevance to Alzheimer's disease. Neurochemistry Int. 2001;39(5-6):381-91. https://doi.org/10.1016/s0197-0186(01)00045-6.
-
11.
Bornemann KD, Wiederhold KH, Pauli C, Ermini F, Stalder M, Schnell L, et al. Aβ-Induced Inflammatory Processes in Microglia Cells of APP23 Transgenic Mice. Am J Pathol. 2001;158(1):63-73. https://doi.org/10.1016/s0002-9440(10)63945-4.
-
12.
Tan RH, Wong S, Hodges JR, Halliday GM, Hornberger M. Retrosplenial cortex (BA 29) volumes in behavioral variant frontotemporal dementia and Alzheimer's disease. Dement Geriatr Cogn Disord. 2013;35(3-4):177-82. [PubMed ID: 23406695]. https://doi.org/10.1159/000346392.
-
13.
Rubio-Perez JM, Morillas-Ruiz JM. A review: inflammatory process in Alzheimer's disease, role of cytokines. The Scientific World Journal. 2012;2012.
-
14.
Giraudon P, Bernard A. Inflammation in neuroviral diseases. J Neural Transm. 2010;117:899-906.
-
15.
Browne TC, McQuillan K, McManus RM, O’Reilly J, Mills K, Lynch MA. IFN-γ Production by Amyloid β–Specific Th1 Cells Promotes Microglial Activation and Increases Plaque Burden in a Mouse Model of Alzheimer’s Disease. J Immunol. 2013;190(5):2241-51.
-
16.
Moreira PI, Zhu X, Nunomura A, Smith MA, Perry G. Therapeutic options in Alzheimer's disease. Expert Rev Neurother. 2006;6(6):897-910. [PubMed ID: 16784412]. https://doi.org/10.1586/14737175.6.6.897.
-
17.
Brown MA, Hural J. Functions of IL-4 and control of its expression. Crit Rev Immunol. 1997;17(1):1-32. [PubMed ID: 9034722].
-
18.
Cho W, Kim Y, Jeoung DI, Kim YM, Choe J. IL-4 and IL-13 suppress prostaglandins production in human follicular dendritic cells by repressing COX-2 and mPGES-1 expression through JAK1 and STAT6. Mol Immunol. 2011;48(6-7):966-72. [PubMed ID: 21277633]. https://doi.org/10.1016/j.molimm.2011.01.007.
-
19.
Shimizu E, Kawahara K, Kajizono M, Sawada M, Nakayama H. IL-4-induced selective clearance of oligomeric β-amyloid peptide1–42 by rat primary type 2 microglia. J Immunol. 2008;181(9):6503-13. [PubMed ID: 18941241].
-
20.
Mocali A, Cedrola S, Della Malva N, Bontempelli M, Mitidieri VA, Bavazzano A, et al. Increased plasma levels of soluble CD40, together with the decrease of TGF beta 1, as possible differential markers of Alzheimer disease. Exp Gerontol. 2004;39(10):1555-61. [PubMed ID: 15501026]. https://doi.org/10.1016/j.exger.2004.07.007.
-
21.
Chao CC, Hu S, Ehrlich L, Peterson PK. Interleukin-1 and tumor necrosis factor-alpha synergistically mediate neurotoxicity: involvement of nitric oxide and of N-methyl-D-aspartate receptors. Brain Behav Immun. 1995;9(4):355-65. [PubMed ID: 8903852].
-
22.
Downen M, Amaral TD, Hua LL, Zhao M, Lee SC. Neuronal death in cytokine-activated primary human brain cell culture: role of tumor necrosis factor-α. Glia. 1999;28(2):114-27. https://doi.org/10.1002/(sici)1098-1136(199911)28:2<114::aid-glia3>3.3.co;2-f.
-
23.
Bliss TV, Collingridge GL. A synaptic model of memory: long-term potentiation in the hippocampus. Nature. 1993;361(6407):31-9. [PubMed ID: 8421494]. https://doi.org/10.1038/361031a0.
-
24.
Li A, Katafuchi T, Oda S, Hori T, Oomura Y. Interleukin-6 inhibits long-term potentiation in rat hippocampal slices. Brain Research. 1997;748(1-2):30-8. https://doi.org/10.1016/s0006-8993(96)01283-8.
-
25.
Vallieres L, Campbell IL, Gage FH, Sawchenko PE. Reduced hippocampal neurogenesis in adult transgenic mice with chronic astrocytic production of interleukin-6. J Neurosci. 2002;22(2):486-92. [PubMed ID: 11784794].
-
26.
Madeo J, Frieri M. Alzheimer's disease and immunotherapy. Aging Dis. 2013;4(4):210-20. [PubMed ID: 23936745].
-
27.
Deverman BE, Patterson PH. Cytokines and CNS development. Neuron. 2009;64(1):61-78. [PubMed ID: 19840550]. https://doi.org/10.1016/j.neuron.2009.09.002.
-
28.
Szczepanik A. IL-4, IL-10 and IL-13 modulate Aβ(1–42)-induced cytokine and chemokine production in primary murine microglia and a human monocyte cell line. Journal of Neuroimmunology. 2001;113(1):49-62. https://doi.org/10.1016/s0165-5728(00)00404-5.
-
29.
Lyons A, Griffin RJ, Costelloe CE, Clarke RM, Lynch MA. IL-4 attenuates the neuroinflammation induced by amyloid-beta in vivo and in vitro. J Neurochem. 2007;101(3):771-81. [PubMed ID: 17250684]. https://doi.org/10.1111/j.1471-4159.2006.04370.x.
-
30.
Yang XY, Wang LH, Mihalic K, Xiao W, Chen T, Li P, et al. Interleukin (IL)-4 indirectly suppresses IL-2 production by human T lymphocytes via peroxisome proliferator-activated receptor gamma activated by macrophage-derived 12/15-lipoxygenase ligands. J Biol Chem. 2002;277(6):3973-8. [PubMed ID: 11726648]. https://doi.org/10.1074/jbc.M105619200.
-
31.
Alvarez A, Cacabelos R, Sanpedro C, Garcia-Fantini M, Aleixandre M. Serum TNF-alpha levels are increased and correlate negatively with free IGF-I in Alzheimer disease. Neurobiol Aging. 2007;28(4):533-6. [PubMed ID: 16569464]. https://doi.org/10.1016/j.neurobiolaging.2006.02.012.
-
32.
Mosmann TR, Cherwinski H, Bond MW, Giedlin MA, Coffman RL. Two types of murine helper T cell clone. I. Definition according to profiles of lymphokine activities and secreted proteins. J Immunol. 1986;136(7):2348-57. [PubMed ID: 2419430].
-
33.
Zhao W, Xie W, Xiao Q, Beers DR, Appel SH. Protective effects of an anti-inflammatory cytokine, interleukin-4, on motoneuron toxicity induced by activated microglia. J Neurochem. 2006;99(4):1176-87. [PubMed ID: 17018025]. https://doi.org/10.1111/j.1471-4159.2006.04172.x.
-
34.
Iribarren P, Cui Y, Le Y, Ying G, Zhang X, Gong W, et al. IL-4 down-regulates lipopolysaccharide-induced formyl peptide receptor 2 in murine microglial cells by inhibiting the activation of mitogen-activated protein kinases. J Immunol. 2003;171(10):5482-8. [PubMed ID: 14607954].
-
35.
Tarkowski E, Andreasen N, Tarkowski A, Blennow K. Intrathecal inflammation precedes development of Alzheimer's disease. J Neurol Neurosurg Psychiatry. 2003;74(9):1200-5. [PubMed ID: 12933918].
-
36.
Song C, Halbreich U, Han C, Leonard BE, Luo H. Imbalance between pro- and anti-inflammatory cytokines, and between Th1 and Th2 cytokines in depressed patients: the effect of electroacupuncture or fluoxetine treatment. Pharmacopsychiatry. 2009;42(5):182-8. [PubMed ID: 19724980]. https://doi.org/10.1055/s-0029-1202263.
-
37.
Zuliani G, Ranzini M, Guerra G, Rossi L, Munari MR, Zurlo A, et al. Plasma cytokines profile in older subjects with late onset Alzheimer's disease or vascular dementia. J Psychiatr Res. 2007;41(8):686-93. [PubMed ID: 16600299]. https://doi.org/10.1016/j.jpsychires.2006.02.008.
-
38.
Tobinick E, Gross H, Weinberger A, Cohen H. TNF-alpha modulation for treatment of Alzheimer's disease: a 6-month pilot study. MedGenMed. 2006;8(2):25. [PubMed ID: 16926764].
-
39.
Meda L, Baron P, Prat E, Scarpini E, Scarlato G, Cassatella M, et al. Proinflammatory profile of cytokine production by human monocytes and murine microglia stimulated with β-amyloid[25–35]. Journal of Neuroimmunology. 1999;93(1-2):45-52. https://doi.org/10.1016/s0165-5728(98)00188-x.
-
40.
Strauss S, Bauer J, Ganter U, Jonas U, Berger M, Volk B. Detection of interleukin-6 and alpha 2-macroglobulin immunoreactivity in cortex and hippocampus of Alzheimer's disease patients. Lab Invest. 1992;66(2):223-30. [PubMed ID: 1370967].
-
41.
Cojocaru I, Cojocaru M, Miu G, Sapira V. Study of interleukin-6 production in Alzheimer's disease. Rom J Intern Med. 2011;49(1):55-8. [PubMed ID: 22026253].
-
42.
Bossu P, Ciaramella A, Salani F, Bizzoni F, Varsi E, Di Iulio F, et al. Interleukin-18 produced by peripheral blood cells is increased in Alzheimer's disease and correlates with cognitive impairment. Brain Behav Immun. 2008;22(4):487-92. [PubMed ID: 17988833]. https://doi.org/10.1016/j.bbi.2007.10.001.
-
43.
Lindberg C, Chromek M, Ahrengart L, Brauner A, Schultzberg M, Garlind A. Soluble interleukin-1 receptor type II, IL-18 and caspase-1 in mild cognitive impairment and severe Alzheimer's disease. Neurochem Int. 2005;46(7):551-7. [PubMed ID: 15843049]. https://doi.org/10.1016/j.neuint.2005.01.004.
-
44.
Bossu P, Ciaramella A, Salani F, Vanni D, Palladino I, Caltagirone C, et al. Interleukin-18, from neuroinflammation to Alzheimer's disease. Curr Pharm Des. 2010;16(38):4213-24. [PubMed ID: 21184660].
-
45.
Ojala J, Alafuzoff I, Herukka SK, van Groen T, Tanila H, Pirttila T. Expression of interleukin-18 is increased in the brains of Alzheimer's disease patients. Neurobiol Aging. 2009;30(2):198-209. [PubMed ID: 17658666]. https://doi.org/10.1016/j.neurobiolaging.2007.06.006.
-
46.
Chen L, Grabowski KA, Xin J, Coleman J, Huang Z, Espiritu B, et al. IL-4 induces differentiation and expansion of Th2 cytokine-producing eosinophils. J Immunol. 2004;172(4):2059-66. [PubMed ID: 14764670].
-
47.
Lugaresi A, Di Iorio A, Iarlori C, Reale M, De Luca G, Sparvieri E, et al. IL-4 in vitro production is upregulated in Alzheimer's disease patients treated with acetylcholinesterase inhibitors. Exp Gerontol. 2004;39(4):653-7. [PubMed ID: 15050302]. https://doi.org/10.1016/j.exger.2003.08.012.